Resumen:
Various metals have been used as battery anodes in electrochemical cells ever since the birth of the batteries with Volta’s pile and in the first commercialized primary (Zn/MnO2, Leclanché 1866) and secondary (Pb/acid, Planté 1859) batteries. Li-MoS2 cells, employing Li metal anodes, with specific energies two to three times higher than both Ni/Cd and Pb/acid cells, were withdrawn from the market due to safety issues related to dendrites growth. Instead, Ca are currently being considered as safer metal anode alternative. [1] Pioneering work by Aurbach et al. in the early 1990’s showed a surface-film controlled electrochemical behavior of Ca metal anodes in electrolytes with conventional organic solvents. [2,3] The lack of metal plating was attributed to the poor divalent cation migration through the passivation layer.
Nevertheless, demonstration of Ca plating and stripping in the presence of a passivation layer [4,5] has paved the way for assessment of new electrolyte formulations with high resilience towards oxidation. However, several challenges remain to be tackled for the development of Ca based batteries.[1,6] Among these, the need for reliable electrochemical test protocols, mass transport limitations and high desolvation energies (due to strong cation-solvent and cation–anion interactions) are implied.[8,9] Here, a systematic investigation of the electrolyte formulation impact on the cation solvation structure and transport is presented.[10] Characterization of the passivation layer formed onto the Ca metal anode in various electrolyte formulations using complementary techniques will be presented allowing for the identification of the most suitable passivation layer components in terms of divalent cation mobility.[11] Finally, recent development on cathode materials will be discussed including promising organic cathode materials.[12]
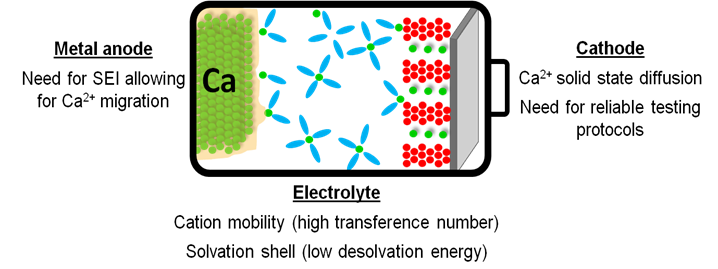
References
1) M. E. Arroyo-de Dompablo, A. Ponrouch, P. Johansson, M. R. Palacín, Chem. Rev. 120 (2020) 6331.
2) D. Aurbach, R. Skaletsky, Y. Gofer, J. Electrochem. Soc.138 (1991) 3536
3) Z. Lu, A. Schechter, M. Moshkovich, D. Aurbach, J. Electroanal. Chem. 466 (1999) 203
4) A. Ponrouch, C. Frontera, F. Bardé, M.R. Palacín, Nat. Mater., 15 (2016) 169
5) D. Wang, X. Gao, Y. Chen, L. Jin, C. Kuss, P. G. Bruce, Nat. Mater. 17 (2018) 16
6) A. Ponrouch, M.R. Palacín, Current Opinion in Electrochemistry 9 (2018) 1
7) A. Ponrouch, J. Bitenc, R. Dominko, N. Lindahl, P. Johansson, M.R. Palacin, Energy Storage Materials 20 (2019) 253
8) D. S. Tchitchekova, D. Monti, P. Johansson, F. Bardé, A. Randon-Vitanova, M. R. Palacı́n, A. Ponrouch, J. Electrochem. Soc., 164 (2017) A1384
9) R. Dugas, J. D. Forero-Saboya, A. Ponrouch, Chem. Mater. 31 (2019) 8613
10) J. D. Forero-Saboya, E. Marchante, R. B. Araujo, D. Monti, P. Johansson, A. Ponrouch, J. Phys. Chem. C 123 (2019) 29524
11) J. D. Forero-Saboya, C. Davoisne, R. Dedryvère, I. Yousef, P. Canepa, A. Ponrouch, Energy Environm. Sci. 13 (2020) 3423
12) D. Monti, N. Patil, A. P. Black, D. Raptis, A. Mavrandonakis, G. E. Froudakis, I. Yousef, N. Goujon, D. Mecerreyes, R. Marcilla, A. Ponrouch, ACS Appl. Energy Mater. doi.org/10.1021/acsaem.3c00969